Understanding the science behind the mobility of IoT devices to improve them to a level Ms.Potts would be ashamed
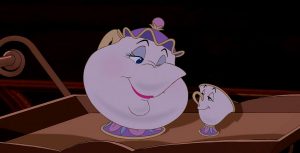
Remember Ms. Potts from Beauty and the Beast? Ms.Potts could speak and probably could whip up a delicious cup of tea just how we like it. One cup of Earl Gray tea with two teaspoons of brown sugar at 80°C? Coming right up!
The Internet of Things (IoT) is simply, where we humans attempt to incorporate such abilities in dumb objects like a refrigerator, toaster, watch, etc by embedding electronic gadgets like sensors in them. These IoT devices gather and share data with other IoT devices within a giant network, where the collected data is analyzed and valuable information is extracted.
Some examples?
Your smartwatch which displays your step count and your smart car which calculates the shortest route to your favorite ramen shop avoiding traffic, are just some everyday examples of IoT devices. Whereas Amazon’s Alexa is an AI that uses the concept of IoT extensively to perform its magic, from streaming music to heating up your leftovers.
It is not surprising that the IoT industry is experiencing rapid growth. Soon enough, our smart refrigerator will nag us to buy milk and our smart scale will not be too far away from blackmailing us.
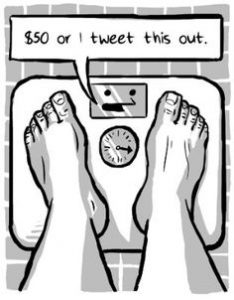
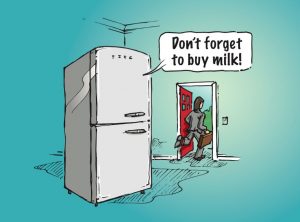
In fact, according to Cisco, there will be 500 billion IoT devices connected to the Internet globally by 2030.
But this expansion in the IoT industry comes with its fair share of problems. In April 2022, a group of scientists from the University of Helsinki decided to conduct research on the mobility patterns of IoT devices in an attempt to provide some solutions to these questions.
What was this study?
This study was the first large-scale measurement study of IoT mobility. A dataset from more than 1.5 million IoT devices in China was used for this study during a period of 36 days. In addition, a signal dataset of 425,000 smartphones from the same cellular network was collected. Then they manually classified these 1.5 million IoT devices into 7 categories based on their function, namely locating devices (such as the GPS devices used to track your Amazon delivery), metering devices (mainly used in intelligent healthcare and smart farming), monitoring devices (such as CCTV cameras which upload real-time video streaming from all around the shopping mall to the security room), portable devices (like your smartwatch), POS or rather Point-of-Service devices (such as ATM machines), industrial terminal devices (mainly manufacturing machines in factories) and vehicles.
They focused on three major problems in their research. Number one, what are the mobility patterns of an IoT device? Number two, what are the differences between IoT and smartphone mobility patterns? Number three, how do mobility patterns defer among different types of IoT devices?
What did they find?
Our brilliant scientists discovered three key points from their research. One, IoT devices move more frequently and use the network more actively in the daytime. Two, the mobility of IoT devices can be predicted. The limits of predictability for smartphones and IoT devices are similar. Three, IoT devices have more ‘important locations’ than smartphones. ( For example, a typical day for me would be going to university for lectures, going to the library, and getting back home. In my case, I have three ‘important locations’ where I take my smartphone: the university, the library, and my apartment. IoT devices have more of these important locations compared to smartphones.)
These results may seem boring or even obvious to us. But the statistics calculated in this study will prove extremely useful.
The question is, how?
For example, currently, IoT devices have access to limited networking resources mainly because they are in direct competition with smartphones for these resources. With the rising popularity of IoT devices, if the means to combat resource scarcity are not met, the quality of user experience will be downgraded.
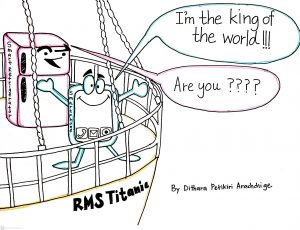
Through statistics calculated by analyzing the mobility patterns of IoT devices, network operators can provide better management of shared network resources and guarantee the best service quality for both types of devices. Therefore, you can gossip with your friends while listening to Taylor Swift’s songs and simultaneously command your future smart coffee maker to make you a latte, without experiencing any delay.
In a nutshell, this research, while not groundbreaking, is an important milestone in improving the IoT industry. Afterall, it is the small things that lead to big changes. When you finally get that nagging refrigerator, you know who to thank.
Author: Dithara Petikiri Arachchige
References:
Xu, D., Wang, H., Li, Y., Tarkoma, S., Jin, D., & Hui, P. (2022). IoT vs. Human: A Comparison of Mobility. https://doi.org/10.1109/TMC.2020.3019988